Revealing the Characteristics of the Three Phases of Titanium Oxide Existing at Different Temperatures – Uncovering the Two Mysterious Phases of Magneli Phase Titanium Oxide - (Press Release)
- Release Date
- 08 Mar, 2010
- BL17SU (RIKEN Coherent Soft X-ray Spectroscopy)
- BL29XU (RIKEN Coherent X-ray Optics)
RIKEN
Japan Synchrotron Radiation Research Institute
Key research findings
• The characteristics of titanium in the high-temperature phase at 154 K (-119°C) or higher are almost the same as those of general metals.
• The intermediate anomalous phase between the high-temperature metal phase and the low-temperature semiconductor phase is clarified.
• Titanium oxide can be used as a material for next-generation fuel cells, by utilizing its advantages of high oxidation resistance and conductivity.
RIKEN (Ryoji Noyori, President) and the Japan Synchrotron Radiation Research Institute (JASRI; Tetsuhisa Shirakawa, President) succeeded in clarifying the three different phases of Magneli phase titanium oxide (Ti4O7), which exist at different temperatures, using the world's highest performance X-ray photoelectron spectrometer*1 at SPring-8 and the world's highest resolution laser photoelectron spectrometer*2 at The Institute for Solid State Physics of The University of Tokyo. This was achieved through a joint research by Shik Shin, leader of the Excitation Order Research Team, Quantum Order Research Group, RIKEN SPring-8 Center (Tetsuya Ishikawa, Director) and a professor of the Institute for Solid State Physics, The University of Tokyo; Munetaka Taguchi, a research scientist in Prof. Shin's team; Tetsuya Ishikawa, chief scientist of the coherent X-ray optics laboratory, RIKEN SPring-8 Center; Hidenori Takagi, a professor at the Graduate School of Frontier Sciences, The University of Tokyo; Haruhiko Ohashi, an associate chief scientist at JASRI; Yasunori Senba, a research scientist at JASRI, and their colleagues. Applications of titanium oxide in new fields, such as hydrophilic and water-repellent materials, have been developed in addition to its use as a white pigment material and in photocatalysts and photoelectrodes. Researchers have been intensively carrying out fundamental and applied research on titanium oxide. In particular, Magneli phase titanium oxides with the composition of TinO2n-1 (n = 3-9) have a unique structure and characteristics, such as a metal-nonmetal transition, metallic properties and antiferromagnetism*3 even at extremely low temperatures. Among the Magneli phase titanium oxides, Ti4O7 has the highest electrical conductivity (2.75 times that of carbon) and exhibits two significant changes in electrical resistance (increases or decreases by three orders of magnitude) when its temperature is changed. It is known that Ti4O7 has three phases, i.e., a low-temperature phase (approximately -133 °C or lower), a high-temperature phase (approximately -119 °C or higher), and an intermediate phase. Therefore, Ti4O7 has been attracting attention both inside and outside Japan as a promising material for next-generation high-efficiency fuel cells that can contribute to a low-carbon society. Thus far, although research on the low-temperature phase is already underway, the electron state and electrical conduction mechanism of the high-temperature and intermediate phases have remained a mystery. The research group determined previously unknown characteristics of Ti4O7 using various types of X-rays. The results indicate that the characteristics of the high-temperature phase are almost the same as those of general metals; furthermore, it was clarified that the intermediate phase between the high-temperature metal phase and the low-temperature semiconductor phase exhibits anomalous characteristics unlike those of metals and semiconductors. This achievement is expected to be an important guideline for the use of titanium oxides, such as Ti4O7, as materials in next-generation fuel cells. The achievements were published online in the American scientific journal, Physical Review Letters, on 8 March 2010, prior to the printed version published on 12 March 2010. Publication: |
<Figure>
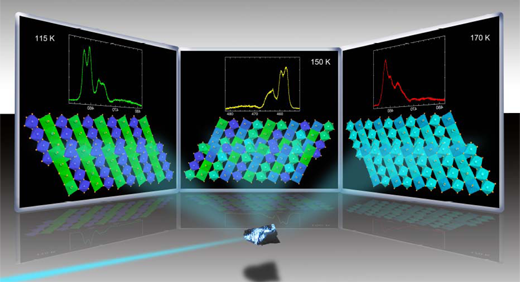
Fig. 1 Three phases of Ti4O7
The three mirrors in the figure show the crystal structure and the relationship between the number of electrons emitted upon the irradiation of a hard X-ray and the electron energy for Ti4O7 in the low-temperature (left), intermediate (middle), and high-temperature (right) phases observed by changing the temperature. In the low-temperature phase, four trivalent Ti atoms (green) and four quadrivalent Ti atoms (blue) are aligned regularly, whereas almost all the Ti atoms (light blue) are uniformly distributed with a valency of 3.5 in the high-temperature phase. In the intermediate phase, Ti atoms with various valencies are aligned in a complicated pattern.
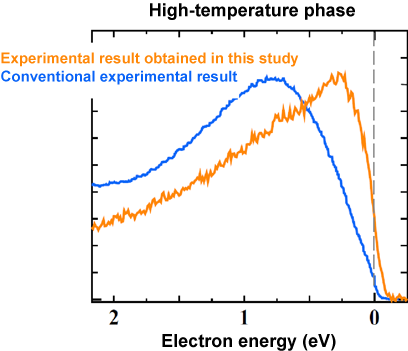
Fig. 2 Soft X-ray resonant photoemission spectrum of
Ti4O7 in the high-temperature phase
The ordinate indicates the number of electrons at corresponding energies. By resonating the energy of a soft X-ray to that of a Ti atom, only the number of 3d electrons of Ti can be increased using the resonance effect of 3d electrons existing in the vicinity of the Fermi level (electron energy of 0). In the conventional experimental result (blue), the spectrum intensity increases gradually from the Fermi level, and no Fermi edge, proof that a substance is a metal, was observed. In this study, a Fermi edge, at which the intensity sharply increases from the Fermi level, was successfully observed in the spectrum by increasing the number of 3d electrons of Ti by the resonance effect.
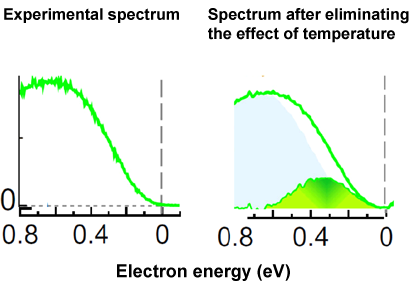
Fig. 3 Laser photoelectron spectrum of
Ti4O7 in the intermediate phase
(Left: Laser photoelectron spectrum with high resolution (5 meV))
In the photoelectron spectrum of Ti4O7 in the intermediate phase, no gap exists in the vicinity of the Fermi level (electron energy of 0).
(Right: Spectrum after eliminating the effect of temperature)
In the spectrum after eliminating the effect of temperature, two components exist: a component of 3d electrons that are firmly bound to Ti atoms (light blue) and a component of 3d electrons that can move freely in the crystals (green).
<Glossary>
*1 X-ray photoelectron spectroscopy
X-ray photoelectron spectroscopy is a method of determining the electron state in a material by examining the relationship between the number of electrons emitted from a sample surface upon the irradiation of an X-ray and the electron energy. This method enables direct observation of the energy distribution of electrons in the material and includes hard X-ray core-level photoemission spectroscopy and soft X-ray resonant photoemission spectroscopy.
*2 Laser photoelectron spectroscopy
Laser photoelectron spectroscopy is a method of determining the electron state in a material by examining the relationship between the number of electrons emitted from a sample surface upon the irradiation of a laser of 6.994 eV (electron volts: a unit of energy) and the electron energy. The main advantages of this method are the markedly high energy resolution compared with that of general X-ray photoelectron spectroscopy and the ability to examine the electron state inside a solid, not only on the surface.
*3 Antiferromagnetism
The spins of neighboring atoms in an antiferromagnetic material are aligned in opposite directions; thus, the material has no overall magnetic properties.
For more information, please contact: Dr. Munetaka TAGUCHI (RIKEN) Dr. Masaki OURA (RIKEN) Dr. Yoshinori NISHINO (RIKEN) |
- Previous Article
- Dr. Hiromitsu Tomizawa Wins 11th Nishikawa Prize of Foundation for High Energy Accelerator Science (Topic)
- Current article
- Revealing the Characteristics of the Three Phases of Titanium Oxide Existing at Different Temperatures – Uncovering the Two Mysterious Phases of Magneli Phase Titanium Oxide - (Press Release)