World's First Realization of Ultrahigh Pressure and Ultrahigh Temperature at the Earth's Center - Finally reaching the Earth's Core
What is the Earth's interior like?
How was the Earth born and how did it reach its current state? The clues to these questions are hidden in the Earth's interior, which has a layered structure consisting of the core, mantle, and crust in increasing order of distance from its center (Fig. 1). This layered structure can be confirmed by measuring the velocity of seismic waves, which are transmitted through the layers when an earthquake occurs. For example, when an earthquake occurs in Japan, seismic waves are transmitted through the Earth's center to Chile, located on the opposite side of the Earth. Because the seismic waves are refracted or reflected at the boundaries of the layers during transmission, changes in the velocity of the seismic waves and the thickness of the layers can be estimated.
Unfortunately, the components of each layer cannot be identified from seismic waves. One method of identifying them is to bore holes into the Earth and sample materials from its interior; however, materials deeper than the crust have not yet been sampled. Another method is to examine rock carried from deep inside the Earth to the surface by magma, a melt found in the mantle*1. Rock containing diamond is considered to be present at depths of greater than 150 km because diamond is produced at a pressure of 5 GPa, which exists at such depths. For this method, however, it is not possible to obtain rock from the mantle originating from depths greater than 200 km. The distance from the Earth's surface to its center is 6378 km, so we can scarcely obtain materials in the Earth's interior.
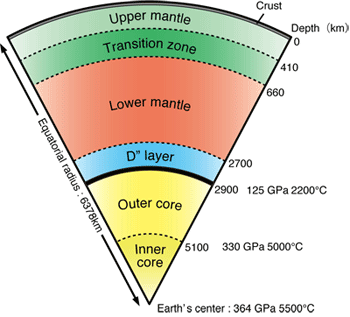
Fig. 1 Layered structure of Earth's interior along with pressures and temperatures
Recreating the environment found deep inside the Earth
Researchers have expended intense effort on experimentally recreating the environment deep inside the Earth and artificially producing materials that can exist in such an environment. The deeper inside the Earth, the higher the pressure and temperature (Fig. 1). The Earth's center has an ultrahigh pressure of 364 GPa and an ultrahigh temperature of 5,500 °C. Researchers worldwide have been continuously devoting themselves to recreating such an environment in their laboratories.
A group led by Kei Hirose, a professor of Tokyo Institute of Technology, holds the world record for the creation of a high-pressure and high-temperature environment. In 2004, the group carried out an experiment to recreate the environment of the D” layer at the bottom of the mantle (i.e., 125 GPa and 2,200 °C or higher), and discovered a completely new mineral, which had a significant impact throughout the field of earth science. In recognition of this achievement, Professor Hirose received the Japan Academy Award, which is given to researchers who have made an outstanding research achievement in their academic field. In 2010, he and his colleagues finally succeeded in realizing the ultrahigh-pressure and ultrahigh–temperature environment at the Earth's center for the first time in the world.
After the first ever synthesis of minerals found in the lower mantle (the upper D” layer) in 1974, it took just 30 years to discover the above-mentioned new mineral. Six years after this discovery, experiments under the high pressure found at the Earth's center became possible; according to Professor Hirose, the progress in recent years has been very rapid.
SPring-8 has accelerated the improvement of high-pressure technologies
The device used for generating pressures as high as 200-400 GPa is surprisingly small and fits in the palm. This device is called a diamond anvil cell, which can be used to apply an extremely high pressure to a sample simply by sandwiching it between two diamond anvils and manually screwing their supports together using a hexagonal wrench (Fig. 2). In addition, ultrahigh temperatures can be simultaneously achieved by irradiating near-infrared rays onto the sample through the diamond.
ccording to Professor Hirose, the smaller the diameter of the diamond tip, the higher the pressure that can be generated. However, it is necessary to cut the tip in an appropriate manner so that it neither deforms nor breaks. In fact, he has shattered many diamonds in his attempt to produce tips with various shapes! After about 10 years of research, he finally succeeded in generating an ultrahigh pressure of 364 GPa. He also modified the device so that it retained the heat generated during laser irradiation, enabling it to simultaneously reach an ultrahigh temperature of 5,500 °C.
Because the ultrahigh pressure and ultrahigh temperature are achieved only in a very small region with a diameter of 20-30 μm (a micrometer is one-millionth of a meter), the samples to be examined must be smaller than this size. How can we analyze such small samples? The solution is to use the X-rays at SPring-8. Because of the small size of the samples, detailed analysis is difficult with X-rays generated from conventional devices. However, the X-rays generated at SPring-8 have high intensity and density as well as the great advantage of allowing the in situ analysis of the crystal structure of samples in a high-pressure and high–temperature environment.
According to Professor Hirose, the pressures achieved in high-pressure experiments remained at approximately 100 GPa for decades until recently. Moreover, even when higher pressures were generated, there were no techniques for analyzing samples under such a high pressure. Thanks to the worldwide development of large synchrotron radiation facilities such as SPring-8, researchers have become further motivated to generate higher pressures, which he believes led to the recent rapid progress in high-pressure technologies.
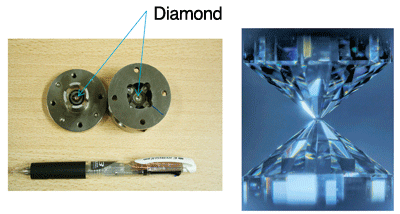
Fig. 2 Palm-size diamond anvil cell used to generate high pressures (left)
The two diamond anvils in the cell are brilliant cut diamonds with a small part of the tip removed (right). When a sample is sandwiched between the tips of the diamond anvils and their supports are screwed together, a very high pressure is applied to the sample.
Clarifying the crystal structure of materials in the Earth's core
Professor Hirose and colleagues, who have enabled experiments to be carried out in the environment found at the Earth's center, are now attempting to clarify how the Earth's core was formed. The Earth's core is mainly composed of iron and consists of a solid inner core and a liquid outer core. In the formation of the Earth, it is considered that the core was initially all liquid but that it crystallized upon cooling over a certain period, resulting in the formation of the solid inner core.
Because technologies for examining liquid samples at ultrahigh pressures and ultrahigh temperatures are not yet fully developed, Professor Hirose first started examining the solid inner core. It is known from the measurement of seismic waves that their velocity differs considerably when they are transmitted in the polar and equatorial directions. This is called seismic anisotropy. In general, anisotropy is observed when crystals are aligned in a certain direction, and anisotropy indicates that a certain type of flow exists in the crystals. Because the dynamics (motion) of the inner core is considered to be related to its creation and growth as well as to the formation of the Earth's magnetic field, scientists have great interest in the flows that exist in the inner core. Although there are still hurdles to be overcome in clarifying this, it is necessary to first determine the crystal structure of iron in the inner core.
Since around 1950, various crystal structures have been proposed for iron in the inner core (Fig. 3). However, even experiments using solid iron were difficult because iron is oxidized and reacts with moisture in air, and its crystal structure easily changes during experiments. In addition, an ultrahigh-pressure and ultrahigh–temperature environment had not yet been realized. Hence, no definitive conclusions were reached. In their study, Professor Hirose and colleagues carried out the world's first experiment in the environment found in the inner core, and revealed that the crystal structure of iron in the inner core is the hexagonal close-packed structure. This finding indicates that iron crystals with the hexagonal close-packed structure, shown in the left of Fig. 3, are aligned parallel to the rotational axis of the Earth.
Professor Hirose said, “In the future, we will further examine the mechanism of the crystal arrangement to clarify the growth and dynamics of the inner core. We will also study the effects of nickel and light elements considered to be present in the inner core on its density and the velocity of seismic waves to determine the chemical composition of the inner core. Using this data, we will also try to determine the chemical composition of the outer core.”
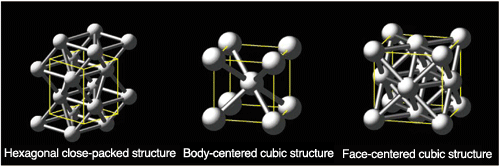
Fig. 3 Previously proposed crystal structures of iron
In the inner core of the Earth, it is considered that iron crystals with hexagonal close-packed structure, shown in the left of the figure, are aligned with the vertical yellow lines parallel to the rotational axis of the Earth.
Column: Poor academic record made him go into geology
Although Professor Hirose has made many noteworthy achievements in earth science, his academic record at Komaba campus of The University of Tokyo was unexpectedly low. He said, “My major was determined by my academic record at the end of the sophomore year. Because of my poor marks, mathematics and geology were the only options for me to pursue in the School of Science. I would never have selected mathematics because my father is a mathematician, so I had no choice but to select geology.” Fortunately, he likes traveling and was interested in geology. “I was blessed by having excellent teachers and senior colleagues in the course of geology in the Department of Earth Science, and studying geology was really enjoyable.”
![]() |
Figure on the cover |
Glossary
*1 Mantle and magma
The mantle is solid rock whereas magma is a liquid, as is often confused. With increasing temperature or decreasing pressure, rock (the mantle) partially melts to form magma. Because the specific gravity of magma near the Earth's surface is smaller than that of the surrounding rock, magma moves upwards towards the Earth's surface because of its buoyancy to form a magma chamber, which cools and solidifies to become plutonic rock or is extruded to form volcanoes.
Interview and original text by Chisato Hata (Sci-Tech Communications)
This article was written following an interview with Professor Kei Hirose at the Graduate School of science and Engineering, Tokyo Institute of Technology.