Electronic Properties
Exploring Electron Movements Responsible for the Manifestation of Functions
SPring-8 Leads the World in Photoelectron Spectroscopy
All materials are composed of atoms, and most physical properties are manifested in accordance to the types of atoms and bonding states. Electrons play a fundamental role in determining the physical properties of a material. Specifically electrons control the electric properties (e.g., electric conductivity and insulation properties, color, reflectance/absorbance of light, thermal conductivity, and magnetic properties). Society depends on various products that utilize properties induced by electrons (Fig. 1). Moreover, environmentally-friendly, energy-saving products have recently been developed; hence, elucidating the electronic properties, which are the bases of these products, is indispensable to create innovative materials. So, various types of spectroscopy have been used to investigate the electronic properties.
Exposing a material to light causes the electrons in the material and light to interact, causing energy to be exchanged, which subsequently emits photoelectrons, Auger electrons, photoions, or new types of light. This circumstance allows the electronic states in a material to be examined by measuring the energies (wavelengths) of the incident light and emitted light/electrons/ions, and calculating the probability of occurrence of such events. This technique is called spectroscopy, and the obtained data are called spectra (Fig. 2).
Among the various types of spectroscopy, photoelectron spectroscopy is the most powerful technique to directly and precisely examine the electronic states in a material. Exposing a material to light allows photoelectric effects, in which electrons in a material receive the energy of the incident light and exit from the material, to be observed. These photoelectron energy measurements are the basis of spectroscopy. Because the energies of photoelectrons reflect the energy states of electrons in a material, researchers have strived to measure the energies of photoelectrons since the early 20th century. However, reliable data from photoelectron spectroscopy was not produced until about 1960 when Kai Siegbahn, who later shared the Nobel Prize in Physics in 1981, was the first to obtain reliable results.
Since the construction of synchrotron radiation factories began in the 1970s, photoelectron spectroscopy has become a common and powerful technique to directly measure electronic states. Moreover, the discovery of high-temperature superconductivity in 1986 greatly improved the resolution to distinguish electronic states, which has enabled more precise measurements, leading to a drastic enhancement in data accuracy. However, as mentioned in Topic 16, Developing High-Resolution Bulk-Sensitive Photoelectron Spectroscopy, conducted by Shigemasa Suga (Professor Emeritus, Osaka University, Japan) and colleagues, such high-resolution photoelectron spectroscopy has been performed mainly in the vacuum ultraviolet ray region, and the information obtained does not reflect the actual electronic states in solids. Fortunately this situation has been rectified with the high-resolution photoelectron spectroscopy performed in the high-energy regions ‾1 keV at SPring-8’s soft X-ray beamline; this spectroscopy has realized high-precision measurements of the actual electronic states within solids as well as in their surface areas. Since then, new techniques have been developed to measure not only the energy of photoelectrons, but also their momentum distributions, providing further information about the electronic states of materials. Through these endeavors SPring-8 has and continues to lead the world in this field.
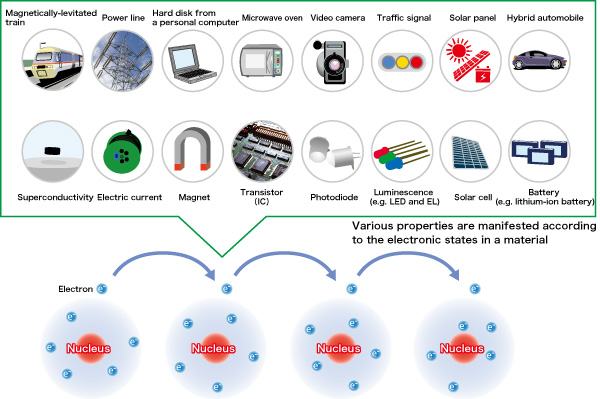
Fig. 1. Various physical properties induced by electronic states.
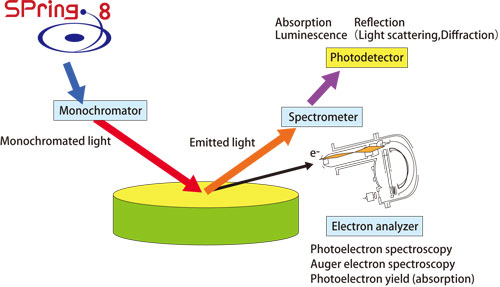
Fig. 2. Schematic diagram of spectroscopy.
Developing New Spectroscopic Techniques to Accelerate Research on Electronic Properties
High-resolution photoelectron spectroscopic experiments using hard X-rays with energy higher than that of soft X-rays, have been successfully conducted at SPring-8 due to the development of a technique called hard X-ray photoelectron spectroscopy (HAXPES). HAXPES can reveal information deep inside a solid. Moreover, HAXPES has expanded the usability of photoelectron spectroscopy using soft X-rays. Photoelectron spectroscopy using soft X-rays used to require ultra-high vacuum conditions and materials with clean surfaces to obtain precise information. However with the advent of HAXPES, researchers can now easily and quickly conduct preparation processes. Consequently, SPring-8 is a global leader in this field as HAXPES can support experiments on various samples, including industrial requests. Due to SPring-8’s advances, researchers in the western world have recently begun to develop a similar experimental apparatus.
Besides photoelectron spectroscopy, other spectroscopic techniques, which are explained in the following sections, have contributed to the research on electronic properties. Absorption spectroscopy measures the amount of absorbed incident light by evaluating samples at various energy values of incident light. Absorption spectroscopy has been applied to a wide variety of research, including the development of various catalysts and battery related materials. Additionally, investigations on the magnetic properties using polarization, which is an advantageous feature of synchrotron radiation, have been vigorously conducted.
Although incident light is absorbed by materials, in some cases this light collides with the electrons in a material and is then emitted. Spectroscopic techniques utilizing this emitted light are indispensable in studies on the electronic states and include X-ray scattering spectroscopy, diffractometry, and fluorometry. By taking advantage of the highly brilliant synchrotron radiation at SPring-8, researchers have conducted unprecedented high-resolution experiments and innovative research projects. These achievements are expected to promote research on superconducting materials and spintronics materials, which are necessary to develop innovative devices.
In the field of spectroscopic research, microspectroscopy, which focuses light to the micrometer or nanometer size, has also advanced. Moreover, in the field of photoelectron spectroscopy, photoelectron microscopy, which magnifies emitted electrons using electron lenses, has achieved a 20-nm1) spatial resolution, and is currently being well utilized to measure small quantities of samples to elucidate physical phenomena specific to microscopic regions (Fig. 3). Furthermore, research on the ultra high-speed changes in the electronic states at the nanosecond2) or picosecond3) level using synchrotron radiation like a strobe flash are advancing.
The spectroscopic techniques described here utilize phenomena induced by direct interactions of light and electrons in a material to obtain information about the electronic states. Additionally, other techniques that examine the electronic states around nuclei using resonant excitation phenomena between nuclei and γ-rays or X-rays are available (e.g., Mössbauer spectroscopy). These other techniques have drastically increased the number of nuclear species that can be spectroscopically measured by resonant excitations at SPring-8 where ultra high-energy X-rays are available. Numerous outstanding achievements have been accomplished through spectroscopic research conducted at SPring-8. However because it is not practical to describe every one, here we describe a select few.
1) nm = 10-9 m
2) nanosecond = 10-9 second
3) picosecond = 10-12 second
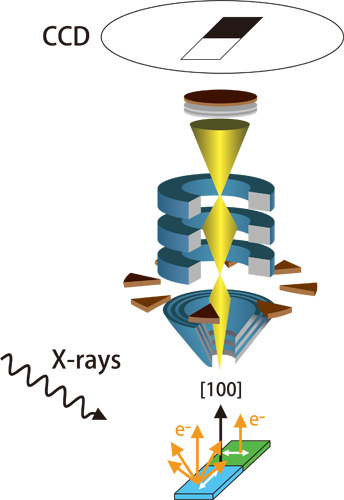
Fig. 3. Schematic diagram of microspectroscopy (photoelectron microscope) in microregions.