Topic 15: Discovering New Phenomena and Quantum States in Extreme Environments
Unexpectedly Changing the Physical Properties under Extreme Pressures and Super-Strong Magnetic Fields
Environments where conditions extremely differ from common environmental conditions are called “extreme environments,” and include environments under ultrahigh temperature or ultrahigh pressures as well as those under super-strong magnetic fields. Because unique phenomena that do not occur under normal conditions exist in extreme environments, many physicists and chemists are attract to research of physical properties under extraordinary conditions. However, creating extreme environments in a laboratory and conducting highly precise experiments in such environments are very difficult. To overcome these obstacles, special devices capable of providing ultrahigh temperatures, ultrahigh pressures, and super-strong magnetic fields have been installed in the beamlines at SPring-8. These devices allow researchers to conduct advanced research on physical properties under extreme environments using highly brilliant synchrotron radiation.
Changing the Physical Properties of Liquids at High Pressure
Phosphorus, which is an important element in biological bodies, has various crystal structures. For example, the structural unit of white phosphorus is composed of regular tetrahedron-shaped molecules where each unit contains four phosphorus atoms, whereas the atoms in black phosphorus are arranged in layers. White phosphorus melts at 44 °C, while black phosphorus does not melt until heated over 600 °C at high pressure. Melted white phosphorus maintains the four-atom regular tetrahedrons. However, the structure of melted black phosphorus was unknown. Thus, Dr. Yoshinori Katayama1) (Senior Scientist), Japan Atomic Energy Research Institute (JAERI)2) and colleagues conducted X-ray diffraction experiments using synchrotron radiation and a high-temperature high-pressure generator at SPring-8 to investigate the transformation of melted black phosphorus at ~1,000 °C by gradually increasing the applied pressure (Fig. 1).
They found that melted black phosphorus drastically transforms the phosphorus arrangement once the pressure exceeds ~1 GPa (GPa = 109 Pa = ~10,000 atm). Under low pressures, the regular tetrahedron-shaped molecules, which are composed of four phosphorus atoms, are randomly arranged, but this arrangement dissolves under high pressures to form a long stretch of interconnected phosphorus atoms, specifically a polymer. This reversible structural transition of melted black phosphorus is induced by pressurization or decompression, and occurs only within a limited pressure variation range of several hundred atmospheres. Moreover, this transition is complete within a few minutes in the fastest case, and the random arrangement and polymer conformation coexist during the transition. From these observations, they believed that this transition is likely due to an increase in a singular phenomenon called the “first-order phase transition of liquid.”
A first-order phase transition is a sudden transition of a material phase accompanied by a drastic density change, which is similar to the phenomenon where graphite is transformed into diamond under high pressures. Several studies have suggested that phase transitions occur in solids as well as some types of liquid. However, a phase transition in liquid had yet to be reported, which inspired Dr. Yoshinori Katayama and colleagues to conduct an experiment at SPring-8 to demonstrate for the first time that a first-order phase transition occurs in neat liquid (melt) under the appropriate temperature and pressure conditions. Their results were published in Nature (January 2000).
Following this study, they then focused on the phase transitions of liquid phosphorus utilizing X-ray transmission imaging (similar to X-ray imaging of human body) and X-ray absorptiometry. In particular, under the conditions of 1,000 °C and 1 GPa, they video-taped the X-ray transmission images of the production and gradual growth of high-pressure liquid-phase spherical droplets. Additionally, they confirmed that the density of the high-pressure liquid phase in the final stage increases by 60% through X-ray absorption measurements. These experiments confirmed that liquid phosphorus exhibits a first-order phase transition, and the world's first example of a liquid-phase transition was published in Science (October 29, 2004). “This research opens a new approach to investigate the structures and phase transitions of liquids, which have not been as thoroughly explored as solids. Like a phase separation between water and oil, if other types of liquid exhibit a phase separation between two phases of the same liquid, then new research fields in fluid dynamics may lead to the development of extraction and transportation of materials,” explains Dr. Katayama with hope.
1) Currently group leader, high-density material research group, Japan Atomic Energy Agency.
2) Currently Japan Atomic Energy Agency (JAEA).
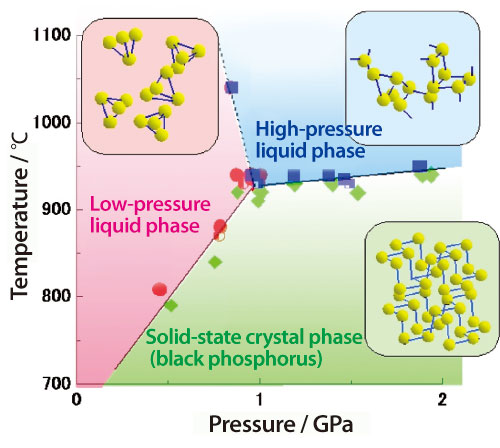
Transition from the low-pressure liquid phase (low-density phase) to the high-pressure liquid phase (high-density phase) occurs with a phase boundary of 1 GPa (GPa = 109 Pa = ~10,000 atm) and a temperature of 900 °C or higher. Tetrahedron structures comprised of four phosphorus atoms in the low-pressure liquid phase are fragmented to form polymer structures in the high-pressure liquid phase. Bonded structures of phosphorus suddenly change at the phase boundary.
Observing Electrons in Special Magnetic Materials Using the World's Most Powerful Super-Strong Magnetic Field
Dr. Hiroyuki Nojiri (Professor, The Institute for Materials Research, Tohoku University, Japan), Dr. Yasuhiro Matsuda (Associate Professor, The Institute for Solid State Physics, The University of Tokyo, Japan), and colleagues revealed the properties of electrons in an europium magnetic material (EuNi2(Si0.82Ge0.18)2) using an X-ray magnetic circular dichroism (XMCD) method, which can examine the magnetic properties of each individual element under a super-strong magnetic field. This super-strong field (40 tesla) is 1,000,000 times stronger than the Earth's magnetic field and is the world's strongest magnetic field.
Suppose that light (an electromagnetic wave) is traveling in space; when the tip of the resultant vector of the electric field (and also the magnetic field) vector components of light describes a circle in a plane perpendicular to the direction of traveling light, the light is called circular polarized light. Circular polarization can be divided into two categories: right (spin: +1) and left (spin: -1). XMCD is the absorption difference between right and left circularly polarized light when X-rays are irradiated onto a magnetized material. Because the magnitude of the absorption difference is proportional to that of material's magnetization, spectroscopy developed based on this phenomenon is used to probe magnetic materials.
All elements preferentially absorb X-rays with an element specific dependent energy, which is called the absorption edge. Thus, XMCD spectroscopic measurements can be performed by adjusting the energy of irradiated X-rays to the absorption edge of each target element. XMCD spectroscopy is suitable to examine the properties of elements, especially the electron states, which are origin of the magnetic properties. “XMCD spectroscopy has been only used to study ferromagnetic materials because compact super-strong pulsed magnetic field generators suitable for materials other than ferromagnetic materials were not available,” comments Dr. Nojiri, who along with his colleagues were the first to observe electrons in europium magnetic materials under a substantially strong magnetic field (40 tesla) by combing their ultra-compact pulsed strong magnetic field generator (Fig. 2) and highly brilliant X-rays at SPring-8.
Europium exhibits an unprecedented property; it suddenly exerts or loses its strong magnetism according to the quantum mechanical mixing state (both position and momentum are uncertain) of two atomic states with different valence values. Although observing the magnetic properties of this material by separating these two ultra-microscopic states was quite difficult, research conducted at SPring-8 has revealed that the magnetic responses of these two states under super-strong magnetic fields completely differ from each other (Fig. 3). Their results demonstrate that the XMCD spectroscopy developed by their research group is a powerful technique to unravel the unprecedented magnetic properties of various materials under super-strong magnetic fields. “I believe that this method can substantially contribute to the effective design and development of new magnetic substances for innovative magnetic memories and magnetic sensors,” hopefully explains Dr. Nojiri. Their research achievements were published in the online edition of Physical Review Letters (July 28, 2009), and Dr. Matsuda received the 30th Honda Memorial Young Researcher Award (2009).
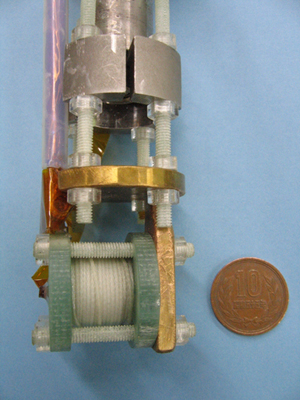
This mini-magnet is composed of 0.7-mm diameter AgCu solenoid coils and reinforced outside by glass fibers. Flanges are made of fiber-reinforced plastics, which are attached onto the head of an insertion device of a refrigerator and cooled to the liquid helium temperature along with samples during the experiments. The 10 Japanese yen coin (diameter ~23.5 mm) is for size comparison.
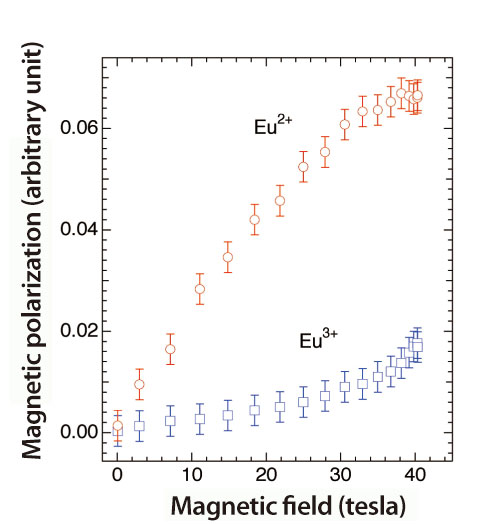
Magnetic field dependence of the magnetic response (magnetic polarization) of Eu for two different valence values (Eu2+ and Eu3+). Magnetic responses of these two electron states completely differ. New techniques have enabled these two states to be separated, which was impossible with previous techniques.
References
1. Y. Katayama, T. Mizutani, W. Utsumi, O. Shimomura, M. Yamakata and K. Funakoshi; Nature, 403, 170 (2000)
2. Y. Katayama, Y. Inamura, T. Mizutani, M. Yamakata, W. Utsumi and O. Shimomura; Science, 306, 848 (2004)
3. Y. H. Matsuda, T. Inami, K. Ohwada, Y. Murata, H. Nojiri, Y. Murakami, H. Ohta, W. Zhang and K. Yoshimura; J. Phys. Soc. Jpn., 75, 024710 (2006)
4. Y. H. Matsuda, Z. W. Ouyang, H. Nojiri, T. Inami, K. Ohwada, M. Suzuki, N. Kawamura, A. Mitsuda and H. Wada; Phys. Rev. Lett., 103, 046402 (2009)