Topic 14: Elucidating the Structure of New Nanoclusters
Drastically Changing the Material Properties through Nanoparticulation
The intrinsic properties of a material remain even if it is broken into tiny particles, especially for solid metals and metallic compounds. However, recent studies have revealed that some metals exhibit unexpected properties, which drastically differ from those in bulk, once they are broken at the nanometer level (nm = 10-9 m). This is analogous to a person showing one aspect of their personality when in a group, but showing other aspects when alone. Synchrotron radiation at SPring-8 contributed to the discovery of this new fact.
Surprise Finding - Gold Nanoparticles Have Magnetism!
Gold is an extremely stable metal and does not oxidize or rust. The melting point of gold, which is 1065 °C under normal conditions, decreases to ~700 °C when it is broken into particles measuring approximately a few nanometers. Although gold was once believed to be chemically inactive and unsuitable for catalysis, it has recently been reported that gold exhibits a high catalytic activity when it is in the form of few-nanometer sized clusters.
The phenomenon where the properties change when the material size becomes sufficiently small is called the size effect. To investigate the size effect in gold (diamagnetic in the normal condition), Dr. Yoshiyuki Yamamoto1) (Assistant Professor, Japan Advanced Institute of Science and Technology) and colleagues have focused on the changes in the magnetic properties of gold nanoparticles. “It is difficult to maintain individual nanoparticles because nanosized particles tend to aggregate,” explains Dr. Yamamoto. To overcome this difficulty, their research group developed a technique to protectively cover the surfaces of nanoparticles with various organic molecules, and successfully maintained individual nanoparticles under stable conditions. Utilizing this technique, they stabilized various sized gold nanoparticles (Fig. 1) and measured the magnetic properties using a high sensitivity magnetic field sensor, a superconducting quantum interference device (SQUID).
Their measurements yielded an unexpected result. Gold nanoparticles with diameters less than 3 nm appeared to become ferromagnetic at an extremely low temperature. However, because SQUID concurrently detects the magnetism from contaminants and the organic molecules covering the nanoparticles, it was difficult to verify that the gold nanoparticles are actually ferromagnetic. To overcome this problem, it was indispensable to develop techniques that enable just the magnetic properties of the gold nanoparticles to be measured.
To this end, Dr. Yamamoto and colleagues employed the Magnetic Materials Beamline (BL39XU) at SPring-8 and measured the magnetism of gold nanoparticles (diameter: 1.9 nm) covered by an organic polymer, polyallylamine hydrochloride, at 2.6 K and a 10 tesla external magnetic field using the X-ray magnetic circular dichroism (XMCD: see Topic 15 for more information) method. This verification measurement is only possible through the combined use of highly brilliant X-rays and the high-precision spectrometer available at SPring-8.
All elements preferentially absorb X-rays with an element-specific dependent energy, which is called the absorption edge. To determine whether gold nanoparticles have magnetism, detailed correlations between XMCD spectrometer data and SQUID data were examined (Fig. 2). The correlations confirmed that gold nanoparticles are actually magnetized. “Due to their size, the ratio of the number of gold atoms in the surface areas to the total number of gold atoms is significantly higher for nanoparticles than that for the bulk. We think this is why gold nanoparticles become magnetized,” shares Dr. Yamamoto. The results of their research, which are expected to contribute to the promotion of magnetism research as well as the development of ultrahigh-density recoding media using ideal noble metal alloy magnetic nanoparticles, were published in Physical Review Letters (September 2004).
1) Currently Associate Professor at Akita University, Japan
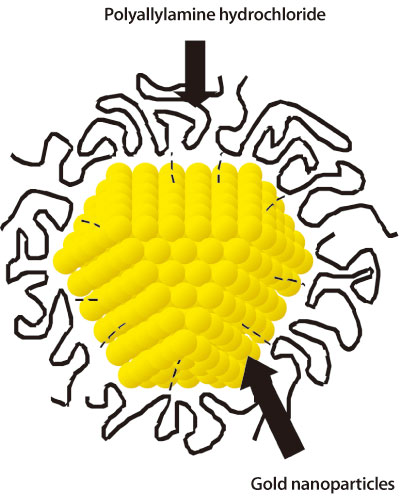
Fig. 1. Schematic view of polymer (polyallylamine hydrochloride) covered gold nanoparticles
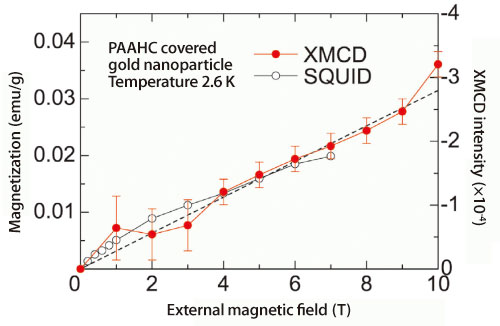
XMCD intensity (red circles) and SQUID (white circles) are correlated, indicating gold nanoparticles are magnetized.
Discovering Nanoparticle Electrolytes Promotes the Development of an All-Solid-State Battery
Electrolytes (ionic conductors) determine the performance of instruments utilizing electrochemical reactions. Similar to the electrolytic solution (sulfuric acid solution) of lead acid batteries, liquid materials typically have high ionic conductivities. Thus, liquid electrolytes are commonly used in button and dry cell batteries. However, these types of batteries require secure metallic packaging, and there are the hazards of abnormal expansion and explosion due to heating or overcharging. From the perspective of stability (nonvolatility), safety (non-explosivity), and ease of manufacturing, including thin-film manufacturing, it is not surprising that the development of highly ionic conductive solid electrolytes is desired.
For this reason, silver iodide (AgI), a solid superionic conductor with a high ionic conductivity equivalent to a liquid, has been receiving much attention as a candidate for the electrolyte in all-solid-state batteries. However, the utility of AgI at ambient temperature is difficult because the superionic conductivity of AgI only appears at 147 °C or higher. Thus, many hurdles remain in the development of solid electrolytes. However, after much time and effort on this topic, Dr. Hiroshi Kitagawa2) (Professor, Kyoto University, Japan) and colleagues hypothesized that, “Employing nanosized particles may work.” Then they put this idea into practice.
First Dr. Kitagawa and colleagues developed a technique to synthesize AgI nanoparticles by mixing, filtering, and drying the solutions of silver nitrate (AgNO3), sodium iodide (NaI), and silver ion conducting poly-N-vinyl-2-pyrrolidone (an organic polymer) under normal temperature and pressure. Next, they developed another technique to selectively produce nanoparticles with a uniform particle size ranging from 10 to 40 nm by optimizing the concentration of each solution and improving the mixing processes. Then in conjunction with Dr. Masaki Takata (Chief Scientist, RIKEN, Japan) and colleagues, they examined the relationship between AgI nanoparticle size and ionic conductivity using the Powder Diffraction Beamline (BL02B2) and a high-brilliance X-ray diffractometer at SPring-8.
They found that the phase transition temperature where superionic conductivity is manifested continues to decrease as a particle size decreases. Moreover, their experiment revealed that the phase transition temperature of ultra-small particles (less than 10 nm) is reduced to ~40 °C, which is ~100 °C less than that of normal AgI particles, while the superionic conductivity is maintained until the temperature decreases to this low phase transition temperature equivalent to room temperature. Additionally, Dr. Kitagawa and colleagues measured the temperature-dependent ionic conductivity of nanoparticles measuring 10 nm, and found that the ionic conductivity of such nanoparticles is more than 100,000 times higher than that of normal AgI particles even at 4 °C after transiting into the normal phase. Furthermore, these nanoparticles are stable under normal atmospheric conditions and maintain their high ionic conductivity even after repeating the heating-cooling cycles.
Prior to their work, systematic studies on the relationship between particle size and the phase transition temperature had yet to be conducted, However, their research provided new techniques to explore superionic conductors. Dr. Kitagawa remarks, “Our research achievements will accelerate the development of all-solid-state batteries (Fig. 3). Sintering at high temperature is required to manufacture inorganic solid electrolytes. In contrast, superionic conductive nanoparticles can be manufactured through simple procedures: solution mixing, filtering, and drying under normal temperature and pressure, and thus, possess great industrial advantages.” Their research achievements were published in the online edition of Nature Materials (May 17, 2009).
2) Also Visiting Professor at Kyushu University, Japan.
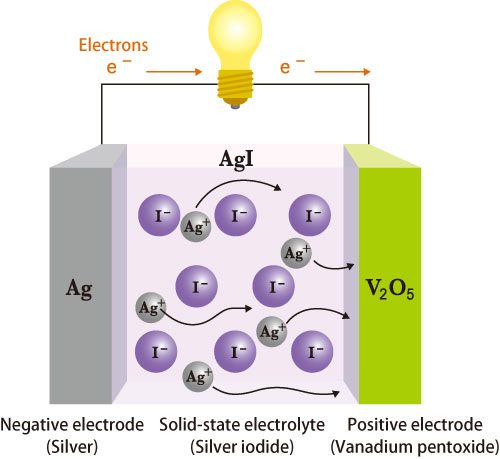
This ideal all-solid-state battery, in which all electrodes and electrolytes are composed of stable materials, does not have problems such as liquid leaks. It is resistant to electric leaks and deformation. Moreover, it is malleable and can be quickly charged/discharged because Ag+ ions move fast in solid-state electrolytes.
References
1. Y. Yamamoto, T. Miura, M. Suzuki, N. Kawamura, H. Miyagawa, T. Nakamura, K. Kobayashi, T. Teranishi and H. Hori; Phys. Rev. Lett., 93, 116801 (2004)
2. R. Makiura, T. Yonemura, T. Yamada, M. Yamauchi, R. Ikeda, H. Kitagawa, K. Kato and M. Takata; Nature Materials, 8, 476 (2009)